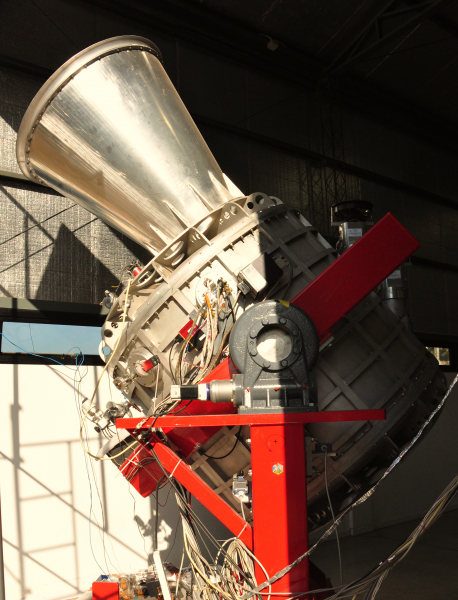
QUBIC is a cosmology experiment designed to study the so-called Inflation Period, when the Universe expanded extremely rapidly immediately after the Big Bang. This observation will be made by measuring the B modes of the CMB polarization. The CMB (Cosmic Microwave Background) is the signal emitted 380,000 years after the Big Bang when light rays were decoupled from the hot matter in the Universe and were able to propagate freely.
Despite the impressive progress made regarding our understanding of the Universe over the last decades [1], several tantalizing questions remain: What is Dark Matter, what is Dark Energy, what happened in the very early Universe? The QUBIC experiment addresses the latter. The inflation paradigm naturally explains the observed flatness and homogeneity and provides a mechanism for generating primordial density (scalar) perturbations. It also predicts tensor perturbations (primordial gravitational waves) that should be imprinted in the CMB polarization fluctuations as odd-parity (curl term), known as B-modes [2] along with the E-modes (even parity, curl-free) which have already been observed. No alternative primordial Universe model can produce primordial B-modes. Their presence would be a non-trivial result concerning quantum gravity because tensor-modes would mean the metric needs to be quantified. As a result, observing primordial B-modes in the CMB is among the main priorities of today’s observational cosmology. The ratio r between tensor and scalar primordial spectra) is directly related to the energy scale at which inflation occurred, providing a direct link to high-energy physics. Further detailed studies of the B-mode signal could provide direct information on inflationary physics [3, 4]. Current best limits on the value of r combine ground-based BICEP-KECK and Planck satellite data, reaching r < 0.06 at the 95% C.L. [5].
We will use the first Bolometric Interferometer, QUBIC, to lower this limit down to r < 0.03 at 95% C.L. (3 years). Such a sensitivity σ(r) = 0.015 (68% C.L.) allows testing a large set of inflationary models.
The small expected amplitude for r (of the order of 0.01), corresponding to a polarization of a few tens (well below the E-mode or temperature fluctuations (respectively 1 and 30 μK)) of nK on top of a 2.7K background brings three major challenges for primordial B-mode detection:
- The sensitivity can be reached using large arrays of background-limited detectors such as bolometers at low atmospheric emission locations (satellites, balloons, dry ground-based sites).
- Instrumental systematics induce spurious polarization that results in mixing between Q and U Stokes parameters inducing leakage of the large E-modes into the small B-modes.
-
Astrophysical foregrounds produce non-primordial B-mode polarization:
- Linearly polarized thermal emission from galactic dust grains has a distinct EM spectrum w.r.t. the CMB. Dust contamination mitigation therefore requires multichroic B-mode measurement.
- Gravitational lensing of E-modes into B-modes caused by large-scale structures peaks at smaller angular scales and will become a serious concern below σ(r)~0.005.
QUBIC :
addressing the three challenges with the novel concept of Bolometric Interferometry
( The concept was initially proposed in 2001 by P. Timbie and L. Piccirillo, both of them members of QUBIC. )
Our objective is the search for the B-mode from the polarization modes Q and U (the two Stokes parameters describing the polarization ellipse of microwave radiation) and combines the advantages of bolometers cooled at very low temperature in terms of sensitivity and those of interferometers in terms of controlling systematic effects of instrumentation and spectrometry. The original idea dates back to the early 2000s and since then, French physicists have played a leading role in its exploration and in exploiting the novelties it brings. After a few initial projects, the QUBIC collaboration was created in 2008. New technical developments at the APC's millimetre laboratory, at the CSNSM-IJCLab in Orsay and in partner laboratories, have enabled the production of cutting-edge detectors, creating a center of excellence with a strong instrumental activity which benefited to a large number of engineers, technicians, researchers and doctoral students, in particular at the site of the APC - University of Paris.
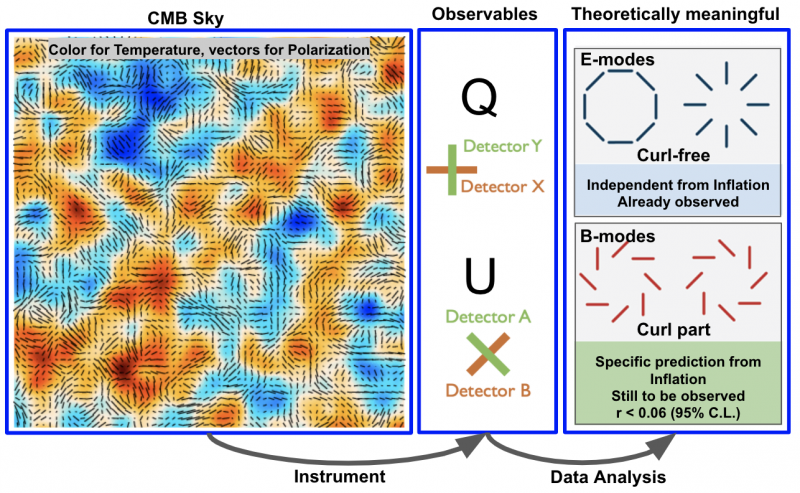
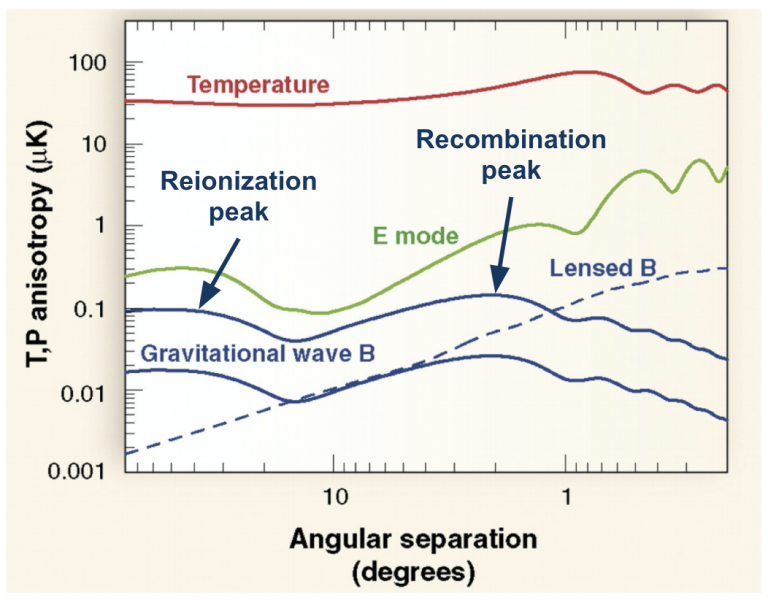
Figure 1. Left: Relationship between sky observables and E and B-modes. Right: Relative amplitude of Temperature (red), E (green) and B-modes (blue) for r=0.1 (excluded) and 0.01. The reionization and recombination peaks are shown with arrows. The lensing from E-mode to B-mode is shown as the dashed blue line.
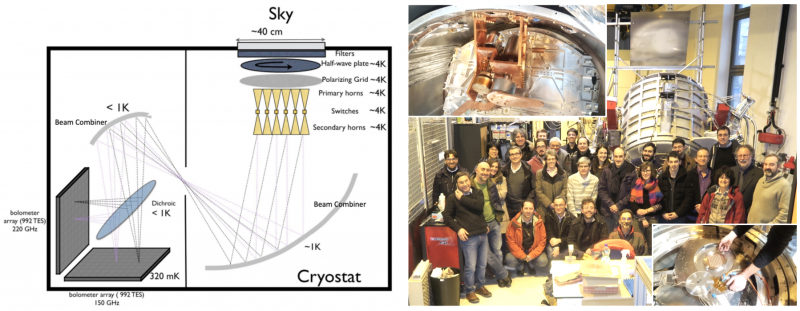
Figure 2. Left: Conceptual design of QUBIC. Right: Picture of the instrument and a part of the Collaboration
After a phase of R&D on subsystems, the QUBIC collaboration began building the Technological Demonstrator (TD) in 2016. It is the same as the Full Instrument (FI) but with fewer detectors (256 at 150 GHz instead of 2048 at 150 and 220 GHz), and with fewer horns (64 instead of 400). The TD was then integrated at APC laboratory starting in 2018 and went through a detailed calibration and testing phase [18] throughout 2019 and 2020. A review, organized in 2020 by CNRS/IN2P3, with INFN, highlighted the innovation from this first ever Bolometric Interferometer, assessed the concept's capabilities as “excellent” and found the spectro-imaging feature “of utmost utility for foreground control” [17].
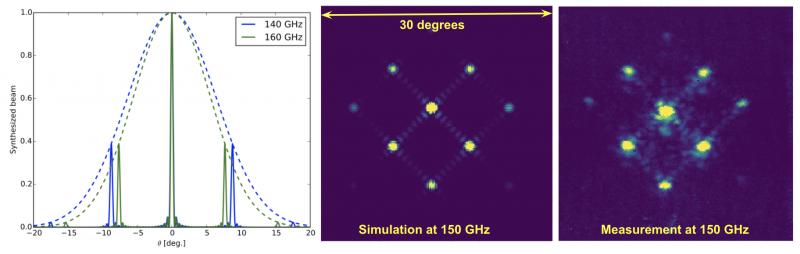
Figure 3. Left: cut across the synthesized beam (at 45 degrees) showing the multiple peaks and their primary beam envelope for two distinct frequencies. Center: 2D simulation of the synthesized beam (predicted in [36]). Right: Measurement of the synthesized beam during the calibration campaign.

Figure 4. Main results of the QUBIC Calibration campaign, together with the synthesized beam shown in Fig. 3. Left: cross-polarization measurement. Center: Individual fringe on the focal plane. Right: Point-source reconstruction.
The next step is the installation of the TD on its 5000m a.s.l. site in Argentina. An integration hall in Salta (3h drive) and a control building in San Antonio de los Cobres (45 min drive) are already operational, while the works on the site are underway. We plan (The schedule presented for QUBIC assumes a relaxation of the COVID19-related constraints by Jan. 2020.) to ship QUBIC in early 2021, assemble and test it in the Integration Hall in Salta (until Aug. 2021) and install it on-site for commissioning in Sept. 2021. We intend to start operations on-sky with the TD in January 2022 (see Figure below for the successive QUBIC scientific phases and upgrades). We plan an upgrade to QUBIC FI after one year of operations with the TD (6 months upgrade). A subsequent upgrade to QUBIC+, an instrument with multi-moded horns after three years operations, is being studied.
Figure 5. Timeline of the three phases with QUBIC upgrades
QUBIC addresses the main B-mode challenges in the following manner :
- QUBIC uses 992 TES with noise 5x10-17 W.Hz-1/2 [18] from a 5000m dry site in Argentina.
-
QUBIC was precisely designed to have low-polarization systematics:
- Low cross-polarization from its optical design (A single polarization is selected at the entrance, any cross-polarization introduced later is irrelevant as detectors are full power.) confirmed by in-lab calibration [18].
- unprecedented control of instrument systematics through the use of “self-calibration” [14].
- Dust foreground: QUBIC uses the novel spectro-imaging technique at 150 and 220 GHz [15, 16]
- Lensing foreground will be considered in Phase 3 through installation of a currently discussed Bolometric Interferometer at the focus of the LLAMA 12m antenna next to QUBIC.
Expected Performance
End-to-end simulations were conducted assuming stable atmosphere and negligible residual systematics after self-calibration [15]. The anticipated performance and scientific objectives are described below:- Phase 1: On-sky demonstration of self-calibration and spectro-imaging with the QUBIC TD. We will focus on bright Galactic regions and measure their SED (spectral energy distribution) using as many as 5 sub-bands within the physical 150 GHz bandwidth of the QUBIC TD. This is illustrated below for one year of data for selected pixels (Note that this will be obtained for all pixels in the map.) - red dots - in both total intensity (a), with 1 deg. pixels, and polarization (b), with 7 deg. pixels. Such high spectral resolution is not achievable with classical imagers. We also plan to perform an upgrade of the QUBIC optics during phase 1 by replacing the 64 horns by the already fabricated and validated 400 horn array and implementing larger mirrors (also already built and measured). This implies replacing the current filters by larger diameter ones. This upgrade would bring a factor 6 sensitivity increase with respect to the simulations shown below.
Figure 6. SED (spectral energy distribution) using as many as 5 sub-bands within the physical 150 GHz bandwidth of the QUBIC TD. One year of data for selected pixels - red dots. (a): total intensity with 1 deg. pixels (b): polarization with 7 deg. pixels
- Phase 2: Constraints on the primordial gravitational waves using QUBIC FI. We will perform deep observations of the clean QUBIC sky region with our two bands (150 and 220 GHz). The anticipated sensitivity combining the two bands and assuming perfect subtraction is σ(r)=0.015 (see (a) below). Dust SED measurement extending to the 220 GHz band will be achievable for each of the patch pixels (see (b) below, 1 deg. pixels). Dust residuals after standard foreground removal as small as 0.7% will be detectable through a frequency dependence (~3σ significance) of the effective r measured in sub-bands, contrary to pure primordial tensor modes (see (c) below). Here again, such foreground control is not achievable with a classical imager, making QUBIC data crucial for the community with future deep B-mode observations.

Figure 7. (a) : Sensitivity combining the two bands and assuming perfect subtraction is σ(r)=0.015
(b): Dust SED measurement extending to the 220 GHz band for a patch pixel of 1 deg.
(c): Dust residuals after standard foreground removal detectable through a frequency dependence of the effective r, measured in sub-bands, contrary to pure primordial tensor modes.
- Phase 3: Upgrade to QUBIC+ and participation in CMB-S4. R&D is ongoing in QUBIC aiming at an order-of-magnitude gain in sensitivity. These include multimode optics (as LSPE/SWIPE or Pixie), hexagonal packing of the horn array instead of the current square packing, lower temperature of the focal plane and optics leading to lower detector and background noise. In parallel, the French IN2P3 community, beyond the current QUBIC collaboration, is forming a consortium to propose a French, possibly European, contribution to the US-led CMB-S4 project (https://cmb-s4.org/) and an upgraded Bolometric Interferometer is a primary candidate currently under discussion. Such an instrument would complement the CMB-S4 instruments with improved capabilities to constrain polarized foregrounds.
Besides direct research, the team intends to develop a significant outreach program in the area around the QUBIC site, particularly targeting scientific education with isolated minorities in the Andes. This will be conducted in collaboration with local scientists, educators and administration and will benefit from the expertise of B. Garcia, co-chair of the Astronomy School Education commission in IAU.
References
[1] Planck 2018 results. VI. Cosmological parameters A&A doi.org/10.1051/0004-6361/201833910 (2020)[2] Zaldarriaga and Seljak, 1997, Phys. Rev. D 55, 1830 (1997)
[3] Abazadjian et al., 2019, CMB-S4 science case, reference design and project plan arXiv:1907.04473
[4] Baumann et al., CMBPol mission concept study, arXiv:0811.3919 (2009)
[5] Ade et al., 2018, Bicep, Keck and Planck, PRD 121, 221301 (2018)
[6] BICEP2 Collaboration; Keck Array Collaboration, PRL, Volume 121, Issue 22, id.221301 (2018)
[7] J.W. Henning et al., ApJ, Volume 852, Issue 2, article id. 97, 31 pp. (2018).
[8] P. Ade et al., Issue 02, article id. 056 (2019).
[9] Th. Louis et al., Issue 06, article id. 031 (2017).
[10] M. Hazumi et al, LiteBIRD Collaboration, JLTP, Volume 194, Issue 5-6, pp. 443-452 (2019)
[11] The QUBIC Collaboration, Series of 8 QUBIC papers submitted to JCAP (special issue on QUBIC, 2020)
[12] Charlassier, Bunn and Hamilton, 2010, A&A, Volume 514, id.A37
[13] Hamilton et al. 2008, A&A Volume 491, Issue 3, 2008, pp.923-927
[14] Bigot-Sazy and Hamilton, 2012, A&A, Volume 550, id.A59
[15] Hamilton, Mousset et al. (The QUBIC Collaboration) 2020, submitted to JCAP, arXiv:2011.02213
[16] Mousset, Gamboa et al. (The QUBIC Collaboration) 2020, submitted to JCAP, arXiv:2010.15119
[17] Review organized by CNRS/IN2P3 with INFN participation
[18] Torchinsky, Hamilton et al. (The QUBIC Collaboration) 2020, submitted to JCAP, arXiv:2008.10056
International website of the project: : http://qubic.in2p3.fr/
QUBIC site at 5000m altitude in Alto Chorillos, near San Antonio de los Cobres province of Salta in Argentina.
The Tuzgle volcano can be seen in the background.
¼ a QUBIC focal plane comprising 256 IOTs cooled to 300mK.
Detection chain developed between APC (Paris), CSNSM-IJCLab (Orsay) and IRAP (Toulouse)
The QUBIC instrument in the integration hall of the APC (Paris)