Waves in thin disks
Spiral density waves are in fact ordinary sound waves, i.e.
compressional perturbations that propagate in the disk, modified by the
various effects that distinguish it from an ordinary fluid:
differential rotation, the epicyclic force, Lorentz force,
self-gravity, and the thin-disk geometry (the disk height is smaller
than the typical wavelengths, giving behaviors similar to waves in
shallow water).
But disks also admit
Rossby waves:
these are well known in planetary atmospheres, where they
propagate perturbations of vorticity (the Great Red Spot of Jupiter can
be viewed as an extreme non-linear form of Rossby waves). In accretion
disks, density waves and Rossby waves are coupled by differential
rotation, exchanging energy and angular momentum: a density wave can
spawn a Rossby wave, and vice-versa. Thus in the
Accretion-Ejection Instability
(AEI) a spiral density wave extracts energy and angular
momentum from the inner region of the disk (this causes
accretion of the gas) and stores them in a Rossby wave near its
corotation radius
(the radius where the wave rotates at the same angular velocity as the
gas), and in this process the spiral may be amplified, making the AEI
an instability. This mechanism also acts in self-gravitating disks (for
galactic spirals) and in disks where the sole force comes from the gas
pressure, although in these cases it is usually stabilizing.
This is where the magnetic field introduces a major
difference, besides
making the AEI unstable: the Rossby wave
represents a twisting motion that is applied to the footpoints of
magnetic field lines threading the disk, and in magnetized plasmas such
a twisting will in turn propagate along the field lines - this is the
nature of
Alfven
waves. Thus the magnetic stresses allows a
significant fraction of the accretion energy and angular momentum,
extracted from the disk, to be re-emitted upward to the corona as
Alfvén waves: this explains the "E" in "AEI", since it turns
accretion into a possible source of energy for jets.
Global modes
Global modes can be analyzed as standing patterns established as
waves, which rotate in the disk at a given frequency
w,
travel
radially back and forth between reflection points. In galaxies, a
spiral wave traveling inward is reflected at the center as an outgoing
wave. In an unmagnetized accretion disk around a black hole,
the
gas reaching the inner edge of the disk (the Marginally Stable Orbit,
or MSO) forms a "plunging region" toward the black hole, and
waves are not expected to be reflected there.
On the other hand various reasons of theoretical or observational
origin, summed up in the mechanism we have called "Magnetic
Floods", have lead us to consider the fate of the vertical magnetic
flux advected with the gas, as it flows toward the black hole. This
magnetic flux must get trapped between the black hole and the disk, and
be contained by a current ring at the inner edge of the disk. No
theoretical or numerical model has yet been able to describe this
configuration, in General Relativistic MHD, when the inner region of
the disk is fully magnetized (i.e. the magnetic pressure is of the
order of equipartition with the gas pressure), and when a strong
magnetic flux has accumulated around the black hole. We believe that it
should be able to efficiently reflect waves.
Spiral waves are characterized by their frequency,

,
and their azimuthal wavenumber,

(
the
number of
arms of the spiral pattern, i.e.

in most
galactic
spirals). It can be shown that they do not propagate in the region of
their corotation radius, where their angular phase velocity,
, is
equal to the rotation frequency of the gas,
W.
When a wave traveling toward corotation reaches this region it is
reflected toward the center of the disk.
1)
Accretion-Ejection Instability:
Thus a standing wave pattern can be established between the inner edge
of the disk and the corotation region, as is classical in such cavities
(e.g. waveguides,
the Solar
interior...). This requires an integral phase condition to be
fulfilled, selecting a discrete set of frequencies.
The following figure describes the basic features of the AEI: it is
formed of a standing spiral pattern between the inner disk edge and
corotation, a standing Rossby vortex near corotation, a spiral wave
emitted outward beyond corotation, and Alfvén waves emitted
from
the Rossby vortex. This qualitative analysis is of course confirmed by
exact, analytical and numerical, derivations in our papers. |
|
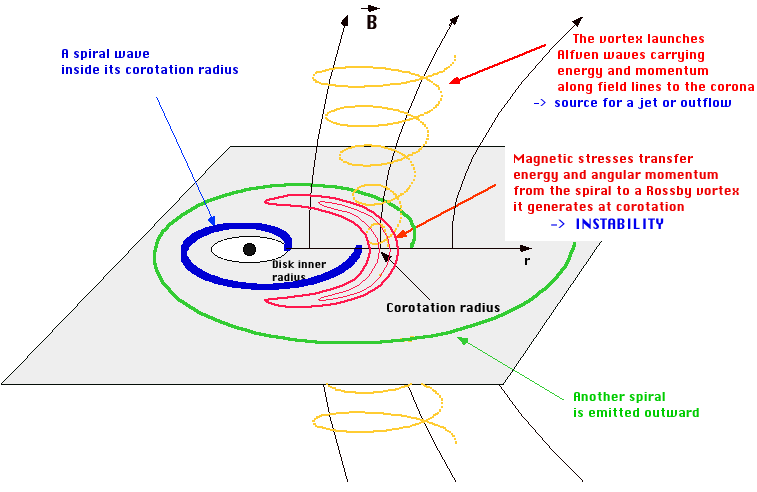 |
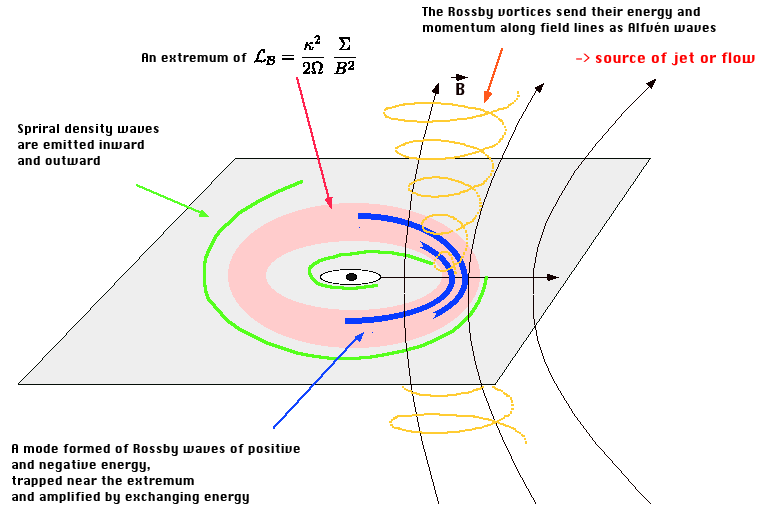 |
|
2)
Rossby-Wave Instability:
If the inner edge of
the
disk ddoes not
reflect waves, another form of global modes can exist if the radial
profiles of density, magnetic field, rotation frequency, etc. create an
extremum of a certain quantity in the disk (we will not describe this
quantity here, but this is similar to the constraint for the
hydrodynamical Kelvin-Helmholtz instabiliy). This can be created by an
extremum of density or magnetic field, but it also occurs naturally,
because of the relativistic rotation curve, just outside the Marginally
Stable Orbit.
Rossby waves can be trapped in this extremum and also establish a
standing pattern. The resulting Rossby-Wave Instability was studied in
hydrodynamical disks by Lovelace and his coworkers. We find it much
more unstable in magnetized disks, and have used this to explain, in
particular, the quasi-periodicity observed during the flares of
Saggitarius A*, the black hole at the center of the
Galaxy. |